Drive systems based on parallel kinematics with electromagnetic and electro-ceramic drives can improve dynamics, resolution and accuracy in applications needing high precision and multiple degrees of freedom.
Stefan Vorndran, Vice President Marketing
Scott Jordan, Director of NanoAutomation Technologies,
Physik Instrumente L.P. (PI)
Motion simulation is often a crucial piece of the design process for evaluating and improving many devices. This can include vibration testing of vehicles and airplanes, response testing of accelerometers and gyroscopes, and testing of image sensors such as those used in high-end cameras and smart phones. Repeatable multi-axis motion simulation can help tweak advanced algorithms in image stabilization systems, and verify the performance of opto-mechanical or electronic stabilizers.
Many emerging fields of structural and active mechanical subsystem analysis, validation and design can also benefit from flexible, multi-degree-of-freedom (DOF) motion mechanisms capable of generating specific motion patterns in real time.
While vibration cancellation has been widely employed in imaging, recently it is also becoming more of an issue in precision machining and manufacturing processes where guiding errors, uneven surfaces and imbalance can have a negative effect on the outcome of parts whose quality is measured in tenths of microns and below. To improve processes at this precision level, tools such as the simulation of motion/unwanted motion, along with its compensation, are steadily gaining in importance.
Hexapod motion simulators vs. mechanical shakers
An established way to simulate motion is to use mechanical shakers or generic stacks of positioning stages. These could sometimes be deployed to simulate simple target motions or approximate a desired mechanical stimulus. However, such setups are inflexible and limited in their capabilities. For maximum efficiency, motion simulators need to move on highly precise, repeatable trajectories in multiple DOF with precisely the same dynamics as the original motion they are trying to simulate.
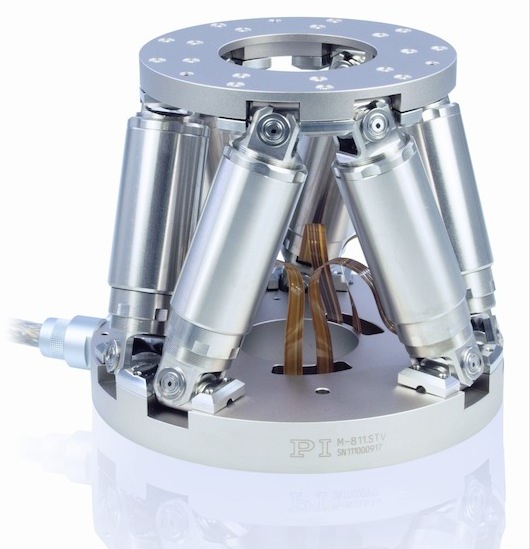
A miniature 6-axis motion simulator with brushless torque motors/screw drive provides high stiffness and motion resolution better than 100 nanometers.
An alternative option for motion simulation is a hexapod. These systems feature a parallel kinematic design with low inertia and symmetrical dynamic performance. They allow precise and exact repeatable motion in the X, Y, Z and pitch, yaw, and roll axes, and a freely selectable center of rotation. High-dynamic hexapods are available together with software toolkits for rapid development of patterned motion generation from the developmental laboratory to the production floor.

In this low-profile, piezo-based hexapod system, Eigenfrequencies in the kHz range enable rapid position control with repeatability in the nanometer range.
These systems can be deployed over a range of applications from simulation and compensation of physiological motions to calibrated oscillation of mechanisms for volume production validation. Hexapods are well suited for such uses due to their signature capability for six-degree-of-freedom motions in a user-defined coordinate system that places the rotational center-point at a desired position in space.
The hexapods’ coordinate system’s origin and orientation can similarly be cast anywhere in space, rather than being fixed by the mechanical configuration of the system. Their controller’s built-in waveform generation is now capable of internal generation of millions of target positions at speeds of up to thousands of points per second, addressing aliasing and generation artifacts. These capabilities are backed up by extraordinarily fast TCP/IP Ethernet interfaces, together with real-time TTL synchronization interfaces, plus available analog inputs and SPI, EtherCAT, and RS-422 interfaces. Two auxiliary motion axes are also integrated for driving additional linear or rotary actuators.
Different mechanical designs
Various drive types are available for highly dynamic simulation of predefined motion. Hexapods with brushless torque motors, and the appropriate mechanical design of the drivetrain and sensor technology achieve accelerations to 2 g. They also provide an inherently stiff drive train.
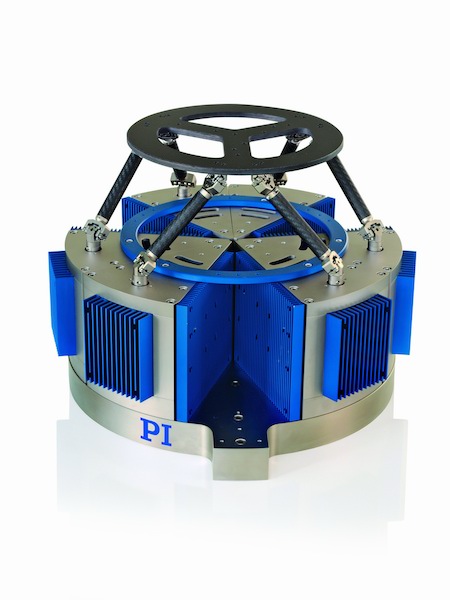
Shown here is a high-dynamic, 6-axis motion simulator based on voice-coil linear drives and flexure joints/guides. Typical areas of application include testing of image stabilization algorithms, and in the medical field for ophthalmology or prosthetics simulating eye motion, eye tracking, and any other human or artificial motion.
Even higher dynamic performance is feasible with voice coil drives (intrinsically soft) such as used in the H-860KMAG flexure guided, direct-drive hexapod, developed by Physik Instrumente (PI). Here accelerations to 4 g with velocities of several hundred mm/sec are possible. The special design includes zero-wear flexure guides and joints, and completely dispenses with rolling or frictional elements. The result is zero-backlash motion without vibrational noise caused by traditional mechanical bearings. Undesired frequency components transferred from the mechanical guiding system cannot influence the measurement.
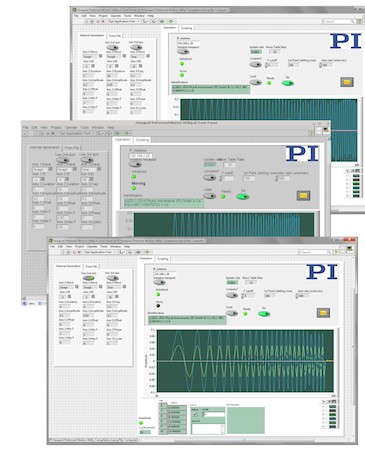
Software for multi-axis patterned motion generation with dynamic hexpaods is available for Windows, Linux, and OSX.
When nanometer-level precision is required and extremely high bandwidth in the kHz range, piezo-based hexapod designs are a suitable choice. The space-saving, parallel-kinematic design allows for a low profile, and small footprint, opening up ultra-precision machining applications where the hexapod compensates guiding errors in real time with nanometer precision. The piezo drives are wear-free and also operate under strong magnetic fields or in a high vacuum.
Piezo scanners for image resolution enhancement/pixel sub-stepping
Low-light conditions pose challenges for imaging applications from astronomy to microscopy. Low light intensity means neither the resolution of the sensor nor the exposure time for changing or moving objects can be freely chosen. Typical applications are fluorescence microscopy, white-light interferometry (OCT in medical technology or general surface structural analysis), or surveillance cameras and cameras for aerial photography. Further fields of application are scanners used to digitize analog data, such as technical drawings, art and paintings.

Examples of highly dynamic piezo scanners for image stabilization/resolution enhancement applications are shown here, with a low-profile XY scanner on the left and a miniature tip/tilt mirror on the right. Both systems are based on parallel kinematics drive designs to provide higher dynamics and precision in a smaller package.
The resolution of digital recording methods is determined by the number of imaging pixels of a CCD or CMOS chip, for example. If one wishes to increase the resolution, the number of imaging pixels must be increased.
There are basically two ways of doing this, both of which are relatively expensive and require considerable effort: a) either increase the size of the recording chip; or b) decrease the size of the pixel. The first case requires a larger recording device and also different imaging optics. In the second case, the light sensitivity decreases with the pixel size. This reduces the separation between image signal and noise signal which, in the end, may even decrease the image quality in spite of the higher resolution.
A method called pixel sub-stepping makes it possible to significantly improve the resolution with relatively little effort.
With pixel sub-stepping, the recording area is moved on a defined path with a defined frequency. This dithering, where the travel is less than the size of a pixel, causes the pixel to be exposed several times on the recording area, producing a virtual pixel multiplier which can significantly improve the resolution. The rest is data processing. The various images produced in this way are subsequently super-imposed to form the final, high-resolution image, a process also known as super resolution.
Because this method is based on motion, a drive is required which meets all the performance criteria for mechanical precision and lifetime, and provides highly reproducible motion of the sensor chip in two dimensions with sufficient linearity. The displacement only needs to be on the order of the pixel size, for example a few tens of micrometers or less, with a wide dynamic range from a few hertz for still images, up to the kilohertz range for video recordings. In addition to planar scanners, tip/tilt scanners can also be used.
In addition to increasing the resolution of a sensor, the scanners can also be operated to actively counteract unwanted motion thereby stabilizing the image and reducing blurring.
Piezo scanners are based on electro-ceramics and precision flexure guiding systems, providing ideal features for these applications. These include solid-state motion, no lubricants required, no wearing parts, and fast response and high resolution in the nanometer range and below.
Physik Instrumente L.P. (PI)
www.physikinstrumente.com
Filed Under: Motors (direct-drive) + frameless motors, Motion Control Tips
Tell Us What You Think!